When the Flying Test Bed A380 aircraft from Airbus hits the skies later this year, it will mark a major milestone in aerospace manufacturing.
That is because the Rolls-Royce Trent XWB-97 engine powering the aircraft will be equipped with the largest civil aero-engine component ever built using 3D-printing techniques.
The component, a front-bearing housing measuring 1.5m across, contains 48 aerofoil-shaped vane components, each of which was produced by 3D printing, or additive manufacturing as it is also known.
Additive manufacturing is an umbrella term that covers a range of processes designed to build up components layer by layer from powdered materials. The technology was initially used by the industry to rapidly produce prototype parts, but it is increasingly being applied to build in-flight production components for aircraft.
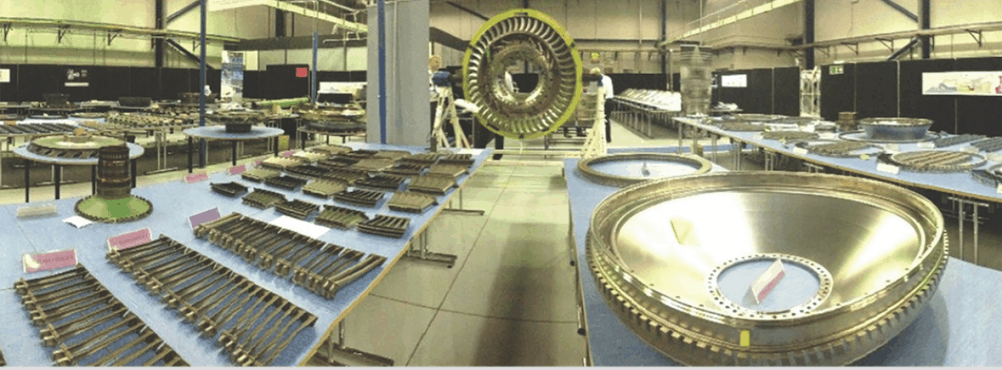
Later this year, for example, GE will begin printing fuel nozzles for the LEAP engine it is developing in partnership with Snecma.
The 3D-printed nozzles will be 25 per cent lighter and five times more durable than the existing nozzles they will replace, which have 20 different parts. They will be produced at a US$50m facility in Alabama, which the company claims will be the first plant in the world to mass-produce 3D-printed aero-engine components.
Boeing, meanwhile, has several hundred types and tens of thousands of 3D-printed parts flying on its aircraft. These include 3D-printed parts on 10 different military and commercial aircraft production programmes, according to Leo Christodoulou, chief engineer for Boeing research and technology, materials and manufacturing technology.
Boeing’s suppliers have been using selective laser sintering to make small, polymer parts for environment control system ducts (ECS) for military aircraft such as the F/A-18 Super Hornet and EA-18G Growler, as well as the company’s commercial aircraft.
“3D printing offers great potential to reduce the cost and weight of aircraft structures and improve the ability of engineers to design parts purely for their eventual function in a vehicle system,” said Christodoulou. “It enables the design and production of monolithic structures, which means converting an assembly and several structures into one piece.”
The company’s metals research is focused on tackling challenges in the supply chain, such as difficult-to-cast or machined parts that are needed in limited quantities.
“We evaluate all categories of additive metals, including large, wire-fed systems, direct metal deposition systems and powder bed systems,” said Christodoulou.
Although Rolls-Royce’s record-breaking front bearing is a prototype rather than a production component, the project has helped the company to understand the challenges of industrialising additive manufacturing technology, said Neil Mantle, head of the centre of competence for additive layer manufacturing at Rolls-Royce. “Influences from new and recycled powder, operating practices and set-ups, multiple machine to machine variation and differing component geometries were all tested and invaluable knowledge gained,” he said.
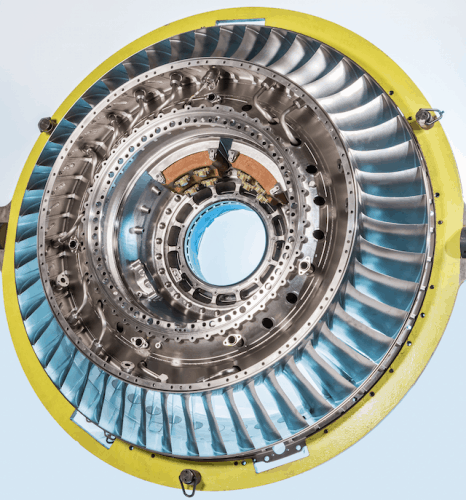
By using additive layer manufacturing for the front bearing, the team was able to spend more time designing and developing the part without the constraint of having to fix its design too early to allow for the long lead time for tooling, said Mantle. “By avoiding this, we reduced the overall manufacturing lead time of the first parts by approximately 30 per cent, while smaller part development continued in parallel using laser based systems.”
GKN Aerospace is also actively investing in additive manufacturing, and is already employing – or will shortly begin employing – a variety of production techniques, according to Dr Rob Sharman, global head of additive manufacturing at GKN Aerospace.
For example, the company uses electron beam melting – a process by which metal components are built up, layer by layer, using a metal powder that is melted by an electron beam – to produce small- and medium-sized titanium components such as fittings and brackets.
Wire deposition techniques, in contrast, are better suited to tasks such as adding features to engine casings, while blown powder deposition can be used to repair and modify high-value components. “Additive manufacturing is a generic term like casting, with multiple processes underneath, and just like casting you use each process depending on the component, the material, and the rate of production,” said Sharman.
“So, generally speaking with small intricate parts with internal channels, I would probably want to use a laser powder bed (machine), but if I’m making a bracket or other small structural part I would want to use electron beam melting because it builds faster, the powder is cheaper, and there is less residual stress,” he added.
When it comes to building structural aircraft components using additive manufacturing, much of the focus to date has centred on making titanium parts as a direct replacement for conventionally produced ones, according to Sharman.
“Particularly for aero-structure components, a lot of additive manufacturing is about making those parts from a near-net [shape] to make a saving on cost, because there is less waste so you’re not throwing all that material away,” he said.
However, as the technology develops, manufacturers will increasingly begin adapting the design of components to take advantage of the specific opportunities the new production processes offer, added Sharman.
Indeed, this is already happening in aero-engine manufacture, where companies are integrating multiple parts into a single complex component, such as GE’s LEAP engine fuel nozzle.
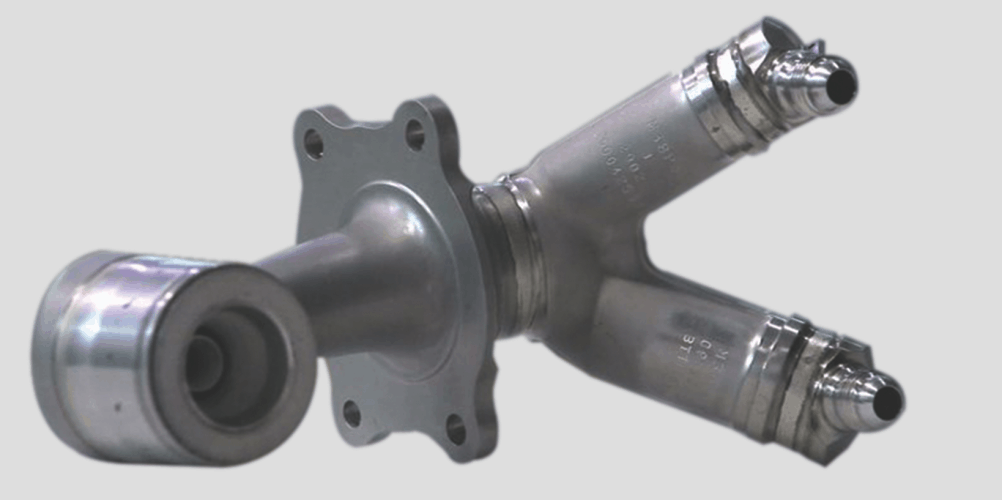
Materials will also be specifically designed for the process. Existing 3D-printed parts are often made from titanium 6Al-4V powder, a widely used alloy originally developed for forgings, said Sharman. “We believe the next stage is tweaking those alloys for other production processes, to make them more receptive to electron beam melting or wire deposition, for example,” he added. “Then you get the full benefits of the process, because you are designing the material for that process.”
To this end, the company is leading a £3.1m research programme, backed by the Aerospace Technology Institute and Innovate UK, to develop titanium powders specifically for additive manufacturing. TiPOW (Titanium Powder for net-shape component manufacture) aims to develop powders formulated and blended to meet the needs of additive manufacturing.
Since the material is generated as the component is being built, aerospace manufacturers will begin tailoring the material’s properties across different areas of the part, Sharman said.This ‘functional loading’ can be used to adapt the microstructure and texture of the component at different points, to meet the varying load across the part. The final step would then be to change the composition of the alloy throughout the part, to meet different loads or requirements, added Sharman.“Everything we currently make looks the way it does because of the way we make it, but nature doesn’t build anything the way we do,” said Sharman. “Now we finally have a process that can help us get closer to the way nature designs and builds things, but we are just at the start of it, and we have a long way to go to emulate that efficiency.”
UK not prepared for climate impacts, says CCC
Perhaps a Longtitude prize to solve railway line problems. "extreme heat causing further disruption through rail buckling and power line...