We’re in a brave new world of engineering innovation, with new inventions and developments enriching our lives every day. Yet some aspects of the devices we depend on have changed little from their inception. It might seem like a contradiction, but sometimes even the most innovative sectors find there are barriers to innovation.
Take, for example, the most visible example of the way technology changed our lives in the last century: the motor car. In many ways, the cars on the roads today are unrecognisable from the contraptions and the early fruits of mass production that trundled down the roads of the 1910s and 1920s. But in others, they have changed very little.
‘People have the perception that cars are basically steel boxes with glass windows, and there’s a good reason for that perception,’ said Prof Richard Dashwood, head of materials and sustainability at the Warwick Manufacturing Group (WMG) and chief technology officer of the new High Value Manufacturing Catapult centre. ‘It is because, largely, they are. Something like 99.9 per cent of all cars on the road are steel-intensive vehicles.’
But the issue of ‘lightweighting’ — reducing the mass of the vehicle — is very much on the minds of automotive manufacturers at the moment. ‘It’s driven by European legislation on CO2 emissions,’ Dashwood said. ‘While you can improve your powertrain and aerodynamics, it’s lightweighting that will give you the biggest CO2 improvement.’
So why, considering the many advances in materials that have taken place over the last century and which have been adopted so enthusiastically by, for example, the aerospace sector, is the automotive industry still so wedded to its original materials?
There are exceptions to this rule. Among the most notable is Jaguar Land Rover, which switched to all-aluminium bodies in 2009, after Jaguar led the way with aluminium construction with the XJ and XK models. Aluminium is, of course, lighter than steel with comparable strength. ‘We didn’t decide to use aluminium because it was new or different,’ said Jaguar’s chief technical specialist for body engineering, Mark White. ‘It is because aluminium delivers significant benefits for drivers.’
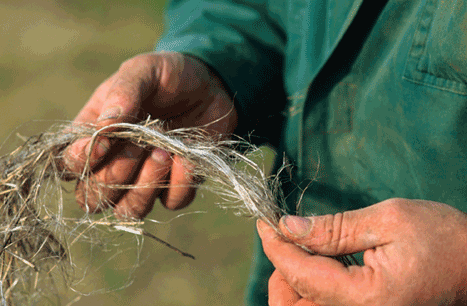
According to Jaguar, the switch to aluminium improves the recyclability of the car, with around half the weight of every Jaguar XJ being made up from recycled aluminium — the equivalent of 12,000 soft drinks cans-worth. There is no difference in quality between new and recycled aluminium, White said. Moreover, 85 per cent of the car is itself recyclable, and it takes just 5 per cent of the energy needed to make a new aluminium sheet to create an identical sheet from recycled metal.
Something like 99.9 per cent of all cars on the road are steel-intensive vehicles
Prof Richard Dashwood, WMG
White also pointed out that aluminium, being lighter than steel, makes the cars less dangerous in crashes. ‘The XJ’s structure carries a lot less kinetic energy into a collision, reducing the amount of energy that has to be absorbed,’ he said.
But switching to aluminium comes at a price, according to Dashwood. ‘If you’re set up to produce cars from sheet steel and steel castings and your supply chain is in steel, then there’s quite a cost associated with making the switch. It’s no small thing to contemplate a switchover; there’s a tremendous amount of knowledge to bring together,’ he said. ‘There are a lot of issues with stamping and pressing aluminium alloys that don’t exist with steel, and there’s an education aspect to make sure your workforce can deal with that. It’s not just the fact that the material is different. All the bonding processes are different as well: steel is spot-welded, aluminium is riveted; the skills needed are completely different.’ Indeed, the Jaguar XJ is held together with some 3,100 self-piercing rivets applied by around 100 robots on the production line; each car also includes around 100m of epoxy adhesive.
Lightweighting is driven by European legislation on carbon emissions. Reducing the mass of the vehicle will give the bigest CO2 improvement
‘It’s a major undertaking, and it’s one that only a high-end producer, such as Jaguar Land Rover or Audi, which also uses aluminium bodies, could contemplate,’ Dashwood said. ‘Having said that, the cost of a body-in-white [unpainted car body] in aluminium is not actually as expensive as you’d think.’
Meanwhile, Dashwood added, the steel industry has upped its game. ‘The steel industry has managed to produce a large range of high-strength materials, which means that manufacturers can lightweight by just going to a thinner range of steel. Eventually you run out of stiffness as you get thinner, so there is a limit. But there’s been enough leeway for most producers to get the properties they need without making the switch to another material.’
Cost is an overriding concern for the industry, Dashwood said. ‘There’s nothing cheaper than a steel shell for automotive — if you move to any other material, it’s going to cost you more. Carbon fibre, of course, is the most expensive of the lot, which is why it’s confined to the highest-value, lowest-volume supercars. But you can build up the skillset and learn on a low-volume, high-value product, and trickle that down to the higher-volume, lower-cost models. And each time you do that, you’ll be more efficient and do it cheaper.’ This, he explained, is the root of Jaguar Land Rover’s strategy.
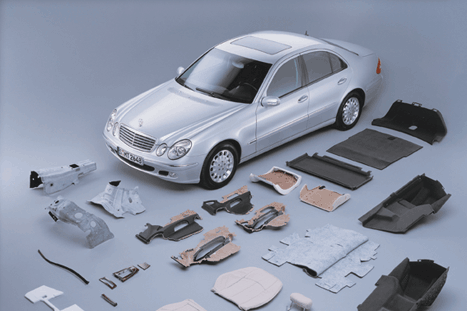
Glass is another heavy component material for most cars, and one which, in the last decade, many observers thought would be replaced. The big hope was in polycarbonate, the tough, transparent polymer that had previously found its largest market segment in the compact disc sector. ‘It’s much lighter, has much better impact resistance and it’s easier to shape because you can injection-mould it, which gives you more options,’ explained Kerry Kirwan, a specialist in sustainable materials and polymer glazing at WMG. ‘But there are various problems. The market penetration has been limited.’
But much as with the steel sector, the glass industry has not been idle. ‘Laminated glass is now lighter and more formable, and there are new ways of chemically toughening glass,’ Kirwan said. ‘The big surge towards polymer glazing has gone away, but it’s a bit up in the air still.’
Headlight lenses are now well established for polycarbonate, but glazing is a different matter, Kirwan said. ‘The transparency of glazing is a safety feature, and because it’s higher up the body, it’s more likely to be scratched — and glass is much more scratch resistant than polycarbonate.’
Apart from lighting, polycarbonate has made its main advances in so-called aesthetic glazing, such as in panoramic roofs. ‘As long as it is not actually something you are looking out of all the time, it’s fine,’ Kirwan added.
Composite components are making headway in volume car production, however, and Kirwan pointed out one definite trend: the replacement of mineral fillers, such as glass fibre, with materials such as hemp. ‘You can burn polymers when you scrap the car, which gives you some energy reclamation, and if the filler is biomass-derived, that means you can burn the composite part as well.’
We didn’t decide to use aluminium because it was new or something different. It was because aluminium delivers significant benefits for driveers
Mark White, Jaguar Land Rover
Hemp parts are surprisingly widespread in the automotive sector, with Ford, General Motors, BMW, Honda and Mercedes all utilising them. Mercedes, for example, varies the fibre it uses depending on where it manufactures: hemp and flax in Germany; coconut in Brazil; and sisal in South Africa. It also uses a fibre derived from banana plants in the underfloor panels of its cars. Known as abaca, this fibre is resistant to rotting and has been used in the Philippines for many years to make ropes, and was the first natural fibre to meet the quality standards for components for the outside of vehicles (other natural fibre materials are used inside cars, such as in door and roof liners, boot panels and so on).
Today, a Mercedes C-class contains some 20 components made from natural material composites. But away from the public roads, some vehicles are appearing that contain a great deal more.
Motorsport is proving to be a good proving ground for new materials, said Kirwan, who recently headed up a project to produce a racing car with the maximum possible amount of naturally derived materials. ‘Motorsport doesn’t have the same safety concerns as the commercial automotive sector, where everything has to pass many stringent tests and be fully certified.’
WMG’s EcoF3 car — which is completely indistinguishable from a standard Formula 3 car in looks, apart from its snazzy vegetable-featuring body decals — contains recycled carbon fibre, polymers derived from carrot and potato starch, flax fibres in the body panels, cashew nut shell fibre in the brake pads, soil bean oil foam in the seat panels, plant oil-based lubricants, a halogen-free wiring loom, polycyclic-aromatic free tyres, and it runs on chocolate-derived biodiesel. The development team claims that it can reach speeds of 145mph, although since chocolate-derived biodiesel isn’t on the approved list of fuels for F3, it can’t actually race. Nevertheless, Kirwan believes that the project could help bring renewable materials technology to the forefront of motorsport design.
One area where it might make an impact on the roads, however, is in electric vehicles. ‘Lightweighting is even more important with an electric powertrain,’ said Dashwood. ‘That is because batteries are so heavy, and therefore you need to reduce weight as much as you can.’
Moreover, with the freed-up design constraints of electric vehicles, manufacturers are no longer tied so closely to the forms and methods of traditional vehicles. With this extra freedom, Dashwood said, they might take the opportunity to look at their products with a fresh eye and, as with their powertrain, rethink the vehicle from the ground up. Maybe your first electric vehicle — whenever that happens — might contain more starch than steel.
Non-metallic engines could offer an alternative to standard iron builds
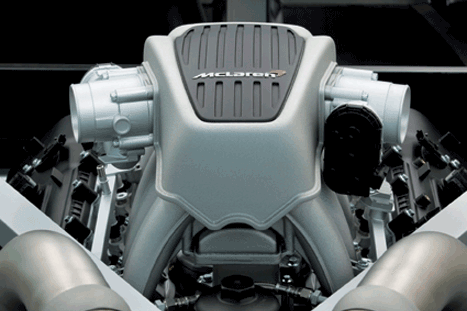
Of all the components of a car, the engine might seem to be the one where material replacement is least likely. However, there has been research into non-metallic engines — and it was more successful than might be thought.
Dating back to the late 1980s, the research was led by Ford, which went into partnership with a US company called Polymotor. The engine was made from a polymer known as Torlon. Based on a chemical system called polyamide-polyimides, Torlon belongs to the same class of polymers as PEEK, a tough engineering material that is still finding applications in high-value niches today. Polyamide-imides can be injection-moulded, before undergoing further curing to impart properties such as strength and heat resistance.
Polymotor’s engine, based on Ford’s then-current 2.3-litre model, consisted of a Torlon block, rods and piston skirts, with metal cylinder sleeves, combustion chamber tops, bearings, valves and seats, and a standard metal crankshaft. It weighed about 70kg (compared with 190kg for a standard iron engine) and produced 318bhp at 11,000rev/min. However, cost issues meant it was never developed.
Cosworth also looked into plastic engines, and in fact raced one in two seasons of US motorsport in the mid-1980s. Weighing 76kg, half of its metal counterpart, it used a polymer engine block, cam cover, timing gears, parts of the air intake, valve assemblies and connecting rods.
Today’s engines are still mainly metal, although the metals involved vary. Cast aluminium is widely used in performance engines, with exotic materials such as magnesium alloys even appearing in the highest-end models. Non-metallics are mainly confined to systems such as the air circuit, where heat resistance is less of an issue. However, cost factors are still the main barrier to further research.
UK research targets toxic forever chemicals
Scaling up may be achievable by layering of channels (as may be done by by PCB heat exchanger designs). In which case, once an optimum channel...