Though no mean feat, decarbonising our power systems is in many ways the easy bit of the energy transition, something the UK is aiming to achieve by 2030. Decarbonising everything else is where things really start getting tricky, not least the industrial processes that will be the engines of the net zero drive.
Heavy industry underpins everything from renewables and EVs to batteries and power transmission, with things like cement, steel, copper and chemicals integral to the decarbonisation pathway. But the production of these core materials is also enormously energy-intensive, and invariably powered by fossil fuels. Heavy industry’s direct CO2 emissions make up for nearly a quarter of the global total, meaning it is simultaneously a huge part of the problem yet fundamental to the solution. Addressing this conundrum is one of the biggest challenges engineers are grappling with today.
What we are looking to do is to decarbonise heavy industry, so the most energy intensive and highest emitting sectors, and doing that in gigaton scale
“What we are looking to do is to decarbonise heavy industry, so the most energy intensive and highest emitting sectors, and doing that in gigaton scale,” Joonas Rauramo, CEO of Helsinki-based Coolbrook, told The Engineer. “From the very beginning, our target has been to make a global impact on CO2 emissions.”
Virtually all heavy industry relies on heat of some description. As it stands, over 90 per cent of industrial heat is derived from burning fossil fuels. Gradually reducing that number is essential, and Coolbrook is confident its electrified heating technology can play a major role.
“Up to now, basically, the high temperature processes have been considered hard to abate and impossible to electrify, and the thought has been that only green hydrogen or CCS can be used,” said Rauramo.
“We bring to the market completely new technology based on turbomachinery through which we can electrify the process heating in steel, cement, chemicals and petrochemicals, aluminium and so forth, and thereby reduce global industrial CO2 emissions by more than 2 billion tonnes annually, representing 30 per cent of industrial CO2 emissions and 7 per cent of all global emissions.”
Coolbrook’s patented RotoDynamic technology is based around a concept originally developed by Soviet rocket scientists to reduce coking during steam cracking of petrochemicals. The electrically powered device harnesses the shock wave phenomenon, whereby the compression of input gases (air, steam, CO2, nitrogen etc) results in a sharp rise in temperature.
Coolbrook’s innovation has been to harness this shockwave phenomenon for industrial heat. It comes in two formats: the RotoDynamic Heater (RDH) delivers high temperature output for applications such as steel and cement production, while the RotoDynamic Reactor (RDR) is designed specifically for electrical steam cracking. Both devices have five turbomachinery stages, each stage raising the temperature by around 200 degrees.
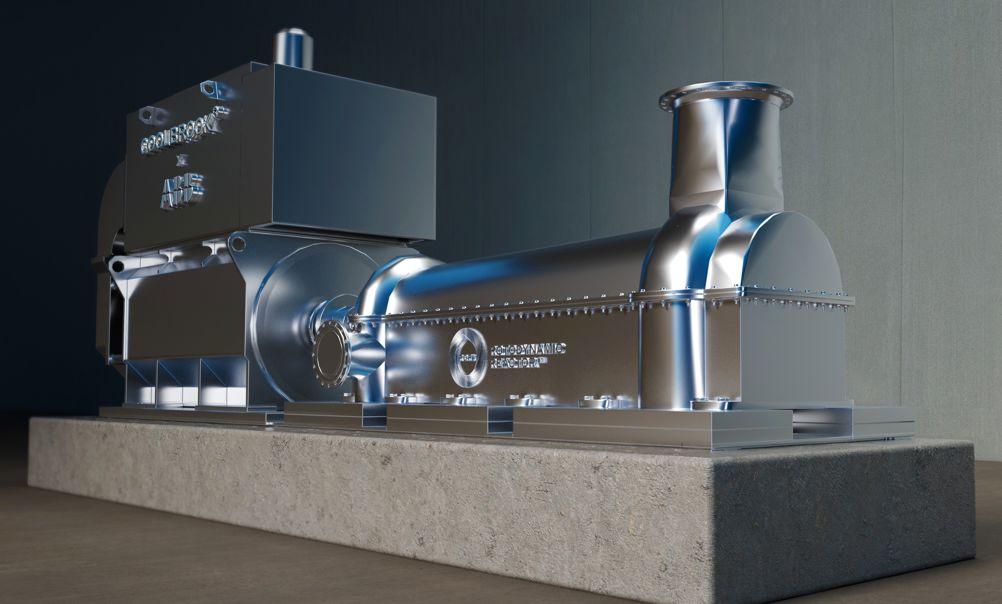
“This is kind of a paradigm shift in turbomachinery,” said Rauramo. “So instead of using a rotating device for pressure generation or for production of electricity, what we look to achieve is very efficient heating.
“Inside the machine, we have a rotating axis driven by the electric motor. And on the axis we have rotating blades. And with the blades, we accelerate the gas, which is to be heated to very high velocity, supersonic velocity, to be more precise.”
The RotoDynamic turbomachinery is powered by ABB drives and motors. Coolbrook and ABB have had a partnership agreement in place since 2022, and Rauramo believes that many synergies exist between the two companies and their respective missions.
“Our technologies and offerings are very highly complementary,” he said. “We have developed what we call a joint offering so that we can offer to the customer a package solution consisting of the required electric infrastructure all the way to the variable speed drive and motor, and then our heater or reactor.”
Inside Coolbrook’s devices, those ABB drives and motors power the rotating axis that generates the supersonic gases. Static diffuser vanes then rapidly decelerate the gas to subsonic speeds. It is this that creates the shockwave, which in turn precipitates the sharp rise in temperature.
“With one stage like this, we can heat air by approximately 200 degrees (Celsius),” said Rauramo. “So having five rotor stages inside the machine, we can heat from ambient to 1,000 degrees.”
If the RotoDynamic technology is paired with input gases that are pre-heated - tapping into waste heat or flue gases, for example - it can deliver output heat of up to 1,700°C. The fully electric process is claimed to be 95 per cent efficient. Paired with green electricity, it has the potential to transform heavy industry, delivering the zero carbon heat the sector so desperately needs.
“Our technology is agnostic to the source of electricity,” said Rauramo. “But of course, in order to achieve the deep decarbonisation of society, we also need to see that the electric grid is at least largely decarbonised.”
Another big selling point is the technology’s relatively small footprint. Industrial heating equipment of this capability generally requires entire buildings dedicated to it. Coolbrook claims the streamlined nature of both the RDH and RDR means they can be dropped in alongside existing industrial processes to meet heat demand where it is needed.
“The machine is extremely compact,” Rauramo. “We can integrate the technology very efficiently to the plants and where the heat is actually required.
“We can go all the way up to 1,700 Celsius degrees, which is roughly 1,000 degrees more than what traditional electric heaters have been able to do.”
Analysis conducted by Coolbrook suggests 1,700°C covers more than 95 per cent of the industrial heat market, giving rise to the headline claims that the technology could single-handedly address around 7 per cent of total global emissions. The company is not shy about its ambitions or the financial rewards on offer.
“The market potential,” said Rauramo, “is unlimited. The industrial heat market is something like 3,000 gigawatts and the total market size well in excess of 1 trillion euros.”
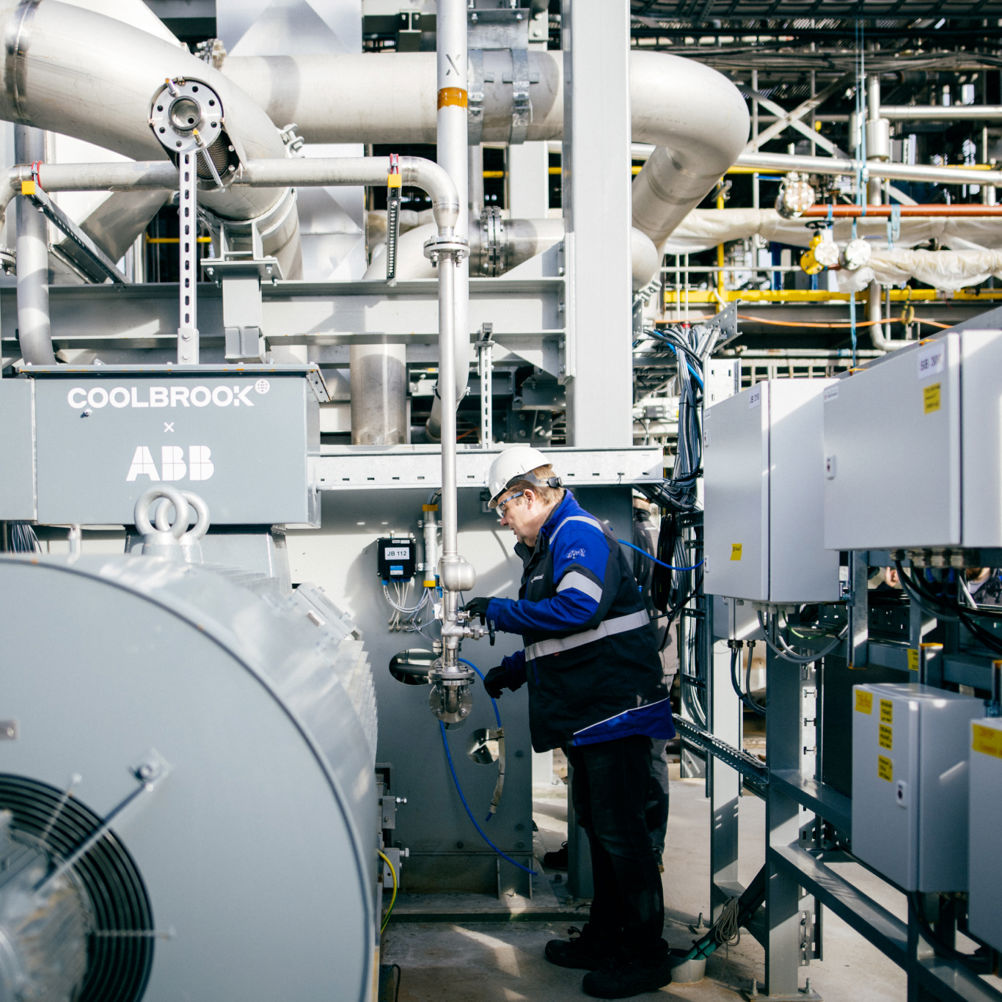
The prize may be huge, but is still some way off. Coolbrook’s first pilot scale plant – a 1MW RDR installation in the Netherlands - has been operational since 2023, successfully demonstrating steam cracking of naphtha and other feedstocks. The company is now on the verge of delivering its first commercial devices, with multiple RDH units expected to be deployed by the end of 2024.
“The first case we foresee to be in steel plants providing high temperature heat up to around 1,000 Celsius degrees, and thereafter the intention is that we move also with the reactor,” said Rauramo. “The target is that we would have at least three units sold by the end of the year.”
Decarbonising heat is undoubtedly a key part of the green industry puzzle, but the net zero drive is vast and complex, touching almost every industrial sector. Producing greener materials for things like sustainable turbine blades, solar panels and batteries is another core strand, with chemical and process engineers to the fore.
Oxford-based start-up FluoRok is making waves in this area, recently raising £7.7 million to scale-up its novel method for manufacturing fluorochemicals. Found in everything from plastics to semiconductors and agricultural products, fluorochemicals are set to play a major role in the energy transition, particularly in Li-ion batteries. According to FluoRok, its patented technology can dramatically reduce the carbon footprint of fluorochemical production.
“FluoRok’s process reacts fluorite with a benign inorganic activator under mechanochemical conditions,” Gabriel Pupo, co-founder and CEO, told The Engineer.
In simplistic terms, FluoRok’s technology mimics the action of a pestle and mortar, generating a new powder that acts as a reagent capable of incorporating fluorine atoms into molecules. The process completely bypasses the creation of hydrogen fluoride (HF) gas - a highly toxic intermediary product in traditional fluorochemical production - while also delivering energy and emissions gains.
“At scale, we expect up to 30 per cent process savings and up to 50 per cent CO2 savings compared to current technologies,” Pupo said.
Today’s market for fluorochemicals is estimated at $24bn. This may be relatively paltry next to Coolbrook’s mammoth figures for industrial heat, but fluorochemicals’ centrality to Li-ion batteries gives them outsized importance in the net zero race. Lithium hexafluorophosphate (LiPF6) makes up around 90 per cent of the cost of the electrolyte in most Li-ion batteries. Growing EV sales and increased general electrification means LiPF6 demand is predicted to rise 10 per cent annually in the coming years.
“More than 15kg of fluorite is needed for every electric car manufactured and this mineral’s reserves are limited,” said Pupo. “It is now on the critical minerals list in both the US and Europe.”
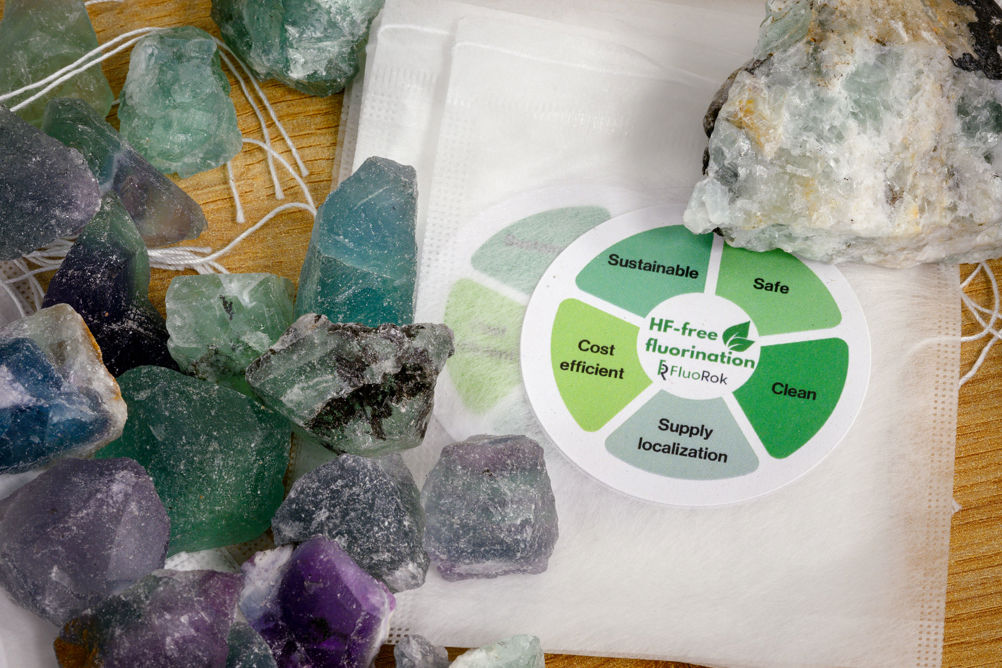
FluoRok’s process aims to address concerns over any fluorite shortage through compatibility with lower grade calcium fluoride (CaF2), meaning recycled fluorite materials can be introduced into Li-ion battery supply chains. For heavy industry to truly go green, this type of circularity will need to be adopted across virtually every aspect of production. Embedding circular practices across supply chains is vital not only to achieve the net zero goal, but also to minimise the environmental impact of industry ramping up to meet that goal.
Recognising the importance of this, automation and energy management specialist Schneider Electric recently partnered with commodities giant Glencore in a bid to improve copper circularity. Copper has a fundamental role in decarbonisation, being an essential component for renewables, electric vehicles, and electricity transmission. It’s also a material present in almost every piece of Schneider Electric industrial equipment. With demand for copper set to increase steeply in the coming years, the partnership with Glencore will help shore up Schneider’s supply, while also developing circularity for both companies’ copper operations.
“Circularity sort of drove the relationship,” John Kwarta, Schneider Electric’s strategic account executive for Glencore, told The Engineer. “When we met with the Glencore recycling team for the first couple of times, we very quickly realised (we’re) both working towards the same mission, which is transitioning to a low carbon economy, but we’re hitting it from exact opposite angles… it’s very complementary, with almost no overlap.
“Very often the most elusive solutions that we struggle with at Schneider are day-to-day business for Glencore. And some of the things that really challenge them the most are things that we do every day at Schneider.”
In some respects, Schneider Electric and Glencore make for strange bedfellows. The French company frequently tops lists of the world’s most sustainable companies, something Schneider is understandably proud of and quick to highlight. By contrast, Glencore’s mining practices around the globe have often attracted criticism, not least its copper and cobalt mine operations in the Democratic Republic of the Congo. But according to Kwarta, Glencore’s decarbonisation ambitions are legitimate.
“Any time we talk about any sort of raw materials, primary producer, commodity, as Schneider we pause, right? We pause and we do our due diligence,” he said.
“If Glencore is going to be, or is becoming, one of our major suppliers of raw materials, obviously we want that material to be as sustainably produced, low carbon as possible. So now that begins to align with our core competencies, which are helping them decarbonise through electrification, help them become more efficient through digitisation, automation, that sort of thing.”
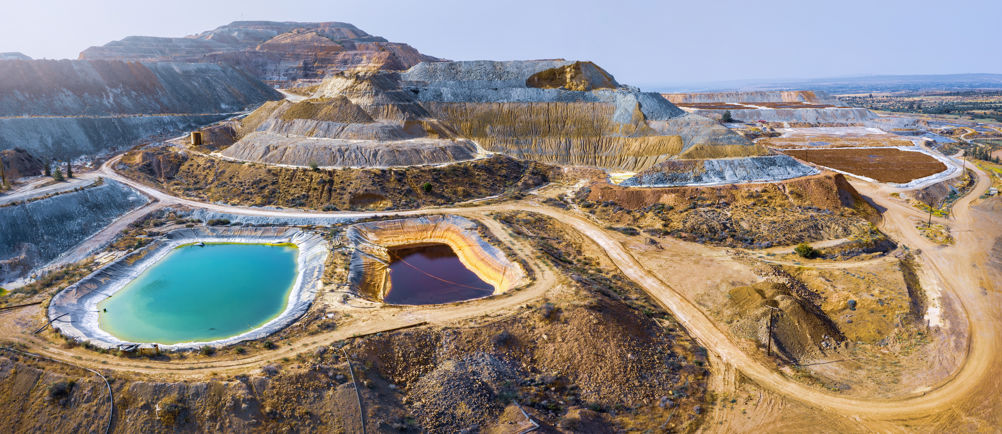
Things like copper wire and pipe are relatively straightforward to recycle, with circular practices well established in most corners of the world. The bigger challenge is dealing with embedded resources in complex devices like batteries and other types of e-waste. It is this complex, multi-material recycling that Glencore and Schneider will target.
“Copper is obviously the point of this story,” said Kwarta, “but the batteries are an equally important second story.
“Glencore’s specialty seems to be more on the elusive things, really focusing on electronic waste, getting not only the copper, but the copper, the platinum, the gold, that sort of thing, out of our products.”
When it comes to primary extraction, Kwarta believes the sector is looking to decarbonise where possible, and the prospect of zero carbon copper at some point in the future is real. Progress is slow however. We may see electrification of certain processes or potentially even some entire sites, but a fully decarbonised mining sector is a long way off. And with net zero set to drive a sustained demand for materials for decades to come, trade-offs are inevitable.
“There’s a lot of debate going on right now about supplying the world with these energy transition materials,” said Kwarta. “And what’s the value of doing a little bit of harm to get those out into the market so we can do greater good, versus maybe holding that back a little bit, slowing the global transition.
“But I will say that it is possible, and that it is likely that zero carbon copper will exist…the question then becomes, do we want to slow our progress down to do that, or is it more important to have those materials available for other segments so we can have maybe a 10 or 100 times benefit?”
Comment: The UK is closer to deindustrialisation than reindustrialisation
"..have been years in the making" and are embedded in the actors - thus making it difficult for UK industry to move on and develop and apply...