Rapid advances in battery chemistry combined with evolving mobility habits are challenging the traditional approach to optimising battery performance. Here, Dr. Doron Myersdorf, CEO of extreme fast charging (XFC) pioneer StoreDot, explains why the battery industry must find new ways to increase the versatility of battery performance at the chemistry level to support a diverse range of driver needs.
The way we traditionally measure battery life cycle performance is based on three key parameters – energy density, charging speed and number of charge-discharge cycles. These variables are closely intertwined, so if you optimise one, the others deteriorate. As such, battery optimisation relies on finding the best combination of these three parameters.
Charging ahead: delivering on the EV dream
UFC FlashBattery recharges drones in five minutes
However, the arrival of new battery chemistries, together with a growing sophistication of battery management systems and evolving driving habits, are changing the game. Suddenly, battery performance is no longer quite so deterministic; instead, it can change over time depending on how the vehicle is driven and charged. This means our mindset also needs to change. We cannot continue to optimise battery performance in the same way as we have previously, as a static function. It needs to be related to the driver’s profile and it needs to be dynamic.
For battery technology developers, the need to identify the habits of the EV drivers of the future is particularly pertinent. While battery management systems give us the flexibility to alter certain parameters over time if the needs of a driver change, the battery chemistry is fixed. Therefore, it is so important to ensure that the chosen chemistry and design of the battery is as closely aligned to the needs of the driver as possible, whether they tend to make short journeys and charge overnight, drive long distances and rely on fast charging points enroute, or their habits lie somewhere in between the two.
New thinking in action
There are many chemistry and electro-chemistry considerations that need to be taken into account to optimise battery formulation and design based on the needs of the driver. This includes establishing the optimum combination of graphite, silicon and/or other metalloids in the anode, determining the right cathode to anode load ratio, and ensuring that the battery’s upper and lower cut-off voltage limits remain within safe boundaries. However, each decision we make has ramifications for other elements of the battery chemistry and design, and so all of this needs to be taken into account during the design phase.
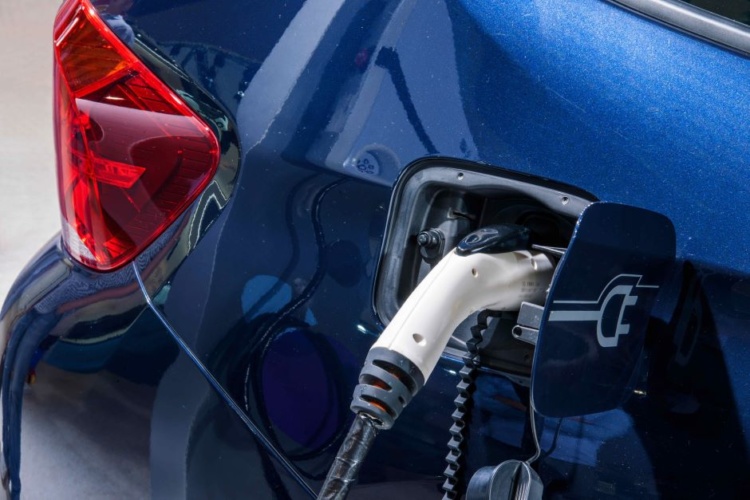
When we developed our XFC technology, one of the key challenges we had to overcome was to manage silicon’s expansion during the fast-charging process. We achieved this by using nanoparticles which are combined in a 3D structure to provide room for the particles to expand without dramatically impacting the overall structure or volume of the anode. When designing batteries to suit a particular operational model, we need to determine not only the best combination of materials to optimise the parameters that are most important to the driver, but also how each combination affects the structure of the anode. So, for example, while batteries optimised for both XFC and slow charging could be silicon dominant, only the XFC battery will require the use of nanoparticles to control the expansion of the silicon during the fast-charging process.
To complicate matters further, the structure of the anode is also influenced by the type of electrolyte and electrolyte additives that are used as part of the SEI layer as the ions move from cathode to anode. As the choice of additives is dependent on whether a battery is optimised for XFC, slow charging, or somewhere in between the two, this also has to be considered as part of the overall anode design.
The combination of materials used in the anode will also affect the upper and lower cut-off voltage limits of the battery. Likewise, we also need to consider how the operational model of the vehicle will impact the cathode to anode load ratio of the battery cell. Establishing the correct ratio is crucial to ensure that in every charge and discharge cycle there is a fully reversible reaction, meaning that all the lithium can be fully transferred back and forth between the cathode and anode. When designing the battery, we can choose to increase the size of either the cathode or the anode by around five per cent to balance the lithium transfer, as each option offers a different point of optimisation. So, in a battery optimised for fast charging, the cathode needs to be slightly larger, whereas in a battery optimised for slow charging, the size of the anode could be increased.
The final destination
If we look further down the road, we can see the last stop in this journey will be offering fully customised EV batteries as standard. While we are currently a long way from achieving this goal, as an industry we need to start thinking differently. It is only by putting drivers firmly in the driving seat that we will successfully unlock the next stage of EV battery development.
Dr Doron Myersdorf, CEO of StoreDot
Water Sector Talent Exodus Could Cripple The Sector
Maybe if things are essential for the running of a country and we want to pay a fair price we should be running these utilities on a not for profit...