Enhancing prosthetic limbs
Human-like muscle fibres made from alloy wires could enhance the movement of prosthetic limbs
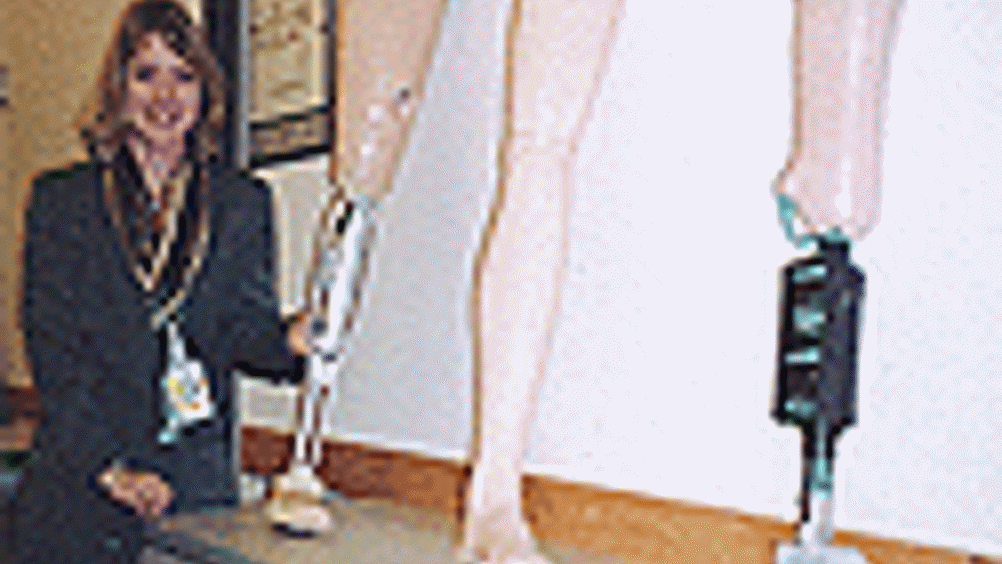
An artificial muscle being developed using shape memory alloy wires that work like natural muscle fibres promises prosthetic limbs that offer more natural movement for amputees.
Chris Harris, professor of neuroscience at
, is leading cross-disciplinary research to develop the muscle based on a model of how the brain and muscles interact to produce movement.
'We're very stereotyped in the way we move,' said Harris. 'If you reach for an object, you do it on a very smooth trajectory. It's to do with how the neural control is organised to optimise accuracy and speed. The brain sends control signals to a local control circuit in your spinal cord and expects certain things to happen.'
The project's vision is to build prosthetic devices or human-like robotic tools that mimic the stochastic, or noise-generating, properties of real muscles.
'When you generate more force, you also generate more noise [irre- levant signals] proportional to the mean force,' said Harris. 'This is a common biological phenomenon, but is not common in artificial systems. The noise originates in the motor neurons, which innervate (supply nerves to) muscle fibres. As you switch on more of them, you get more noise, which makes actions less accurate. The only way to overcome that is to go slower, like the difference between slowly picking up a pin from a table or just quickly touching the table. Speed and accuracy is a trade-off that is unique to biology, and the brain has evolved to expect that trade-off.
Register now to continue reading
Thanks for visiting The Engineer. You’ve now reached your monthly limit of news stories. Register for free to unlock unlimited access to all of our news coverage, as well as premium content including opinion, in-depth features and special reports.
Benefits of registering
-
In-depth insights and coverage of key emerging trends
-
Unrestricted access to special reports throughout the year
-
Daily technology news delivered straight to your inbox
UK Enters ‘Golden Age of Nuclear’
The delay (nearly 8 years) in getting approval for the Rolls-Royce SMR is most worrying. Signifies a torpid and expensive system that is quite onerous...